Your Brain on Pain
- Jeanette Luna
- May 21, 2024
- 12 min read
by Sloane Goldberg
art by Qingyang Meng
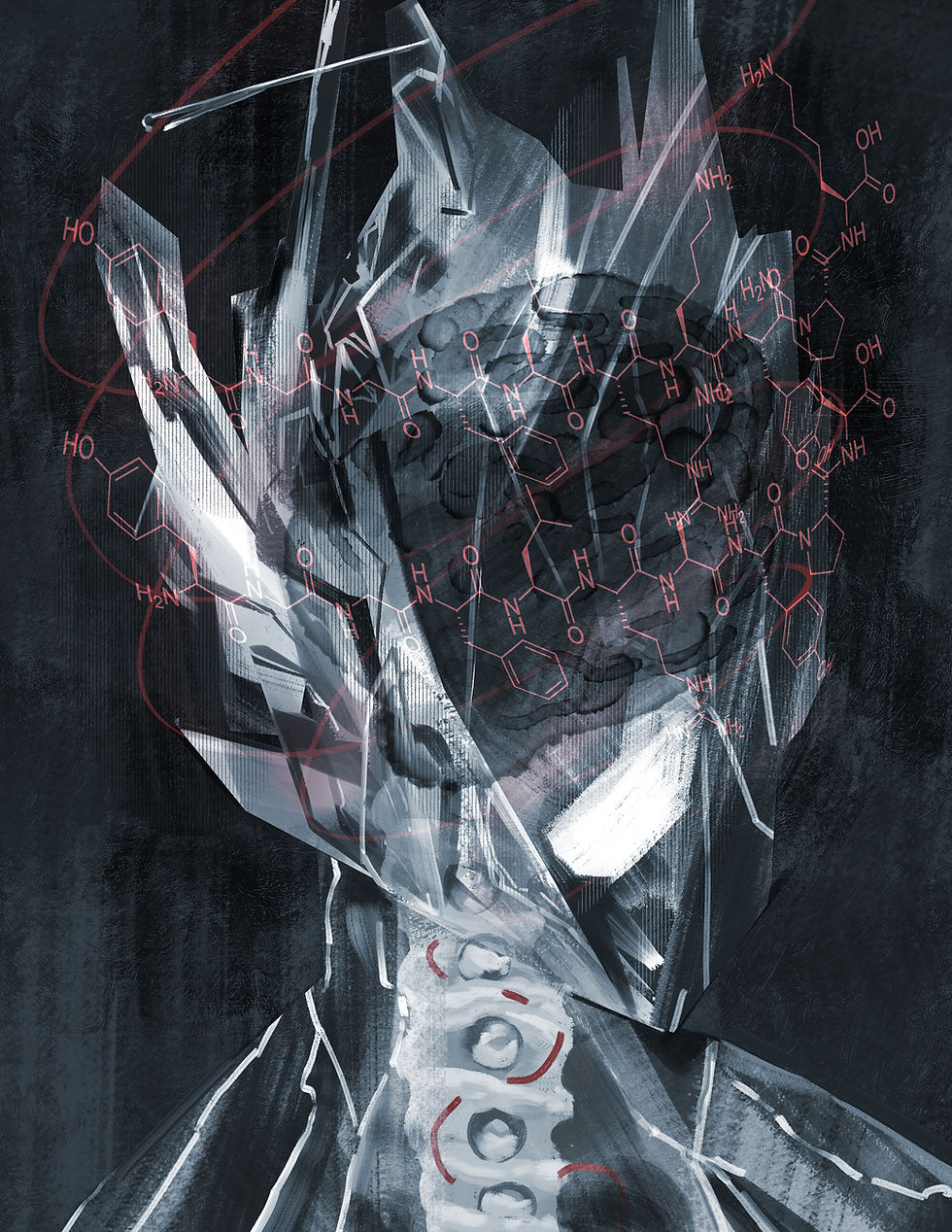
Everything in life revolves around sensations that cycle between pleasant and painful. A hot bath is soothing, but if you increase the temperature by 20 degrees? Painful. Walking is enjoyable, but stubbing your toe on the edge of the couch? Painful. Jalapeño salsa is tasty, but subbing in ghost peppers? Painful. In fact, pain is one of the strongest environmental learning cues we have. Young children learn not to touch hot stoves because they’ll always remember the unexpected pain that shoots through their fingertips after brushing the burner. Even from a philosophical standpoint, we learn to avoid emotions like embarrassment or heartbreak because those feelings are uncomfortable and can even register as physically painful. However, these sensations are not consistent; a bee sting to one person is a nuisance, but to another, it can be insanely painful. What does it mean to have a high pain tolerance, or to push through the pain? How do our brains interpret painful sensations, and why is this interpretation consistently inconsistent?
Though pain is, well, painful, it has an evolutionarily important role in keeping us alive [1]. Individuals born with mutations in pain receptors that inhibit their ability to feel and understand pain often die before adulthood because sensing pain is an important part of staying alive. In these cases of what is called “congenital analgesia” (lack of pain from birth),

placing your hand on a hot stove will still burn your skin, however, you wouldn’t feel the searing pain that most people would expect (Nagasako et al., 2003). It might seem nice to avoid the painful parts of life, like banged elbows, paper cuts, or burns from a hot stove, but those signals are necessary to live. People with congenital analgesia often incur injuries most people wouldn’t even consider, like biting your tongue off or breaking a bone without noticing. Some get hurt or even die doing dangerous activities like jumping off a roof or doing backflips on the lawn. Even though they may fall and crash into the ground, the event still doesn’t register as painful, and therefore doesn’t register as dangerous. Lack of pain doesn’t prevent injury; it prevents learning that certain actions are harmful and must be avoided [1].
The way our brains and bodies deal with pain remains an area of active research. At the beginning of the 20th century, researchers identified specialized pain receptors called nociceptors, which are located in nerve endings that send signals through the skin [2]. These receptors respond to three kinds of triggers: mechanical stimuli like pressure or pinching (stubbing your toe on the couch, breaking your ankle), heat or cold (getting in a scalding bath or stepping barefoot into the snow), or chemical signals (eating ghost peppers or having an allergic reaction) [2].
Nociceptors only respond to sensations that reach a “noxious level” of stimulus [3]. For example, stepping out into brisk fall air will activate thermoreceptors, the receptors that respond to temperature, but falling into a frozen pond will activate nociceptors. At the most general level, these signals travel from the periphery into the central nervous system, transmitting signals from the skin or muscles to the spinal cord. This information is then relayed to the brain and interpreted in higher-level brain areas like the thalamus and the cortex. Because these signals reach important cortical areas so quickly, it is clear that these receptors exist to alert the brain to evolutionarily dangerous stimuli. Broken bones, allergic reactions, burns, and frostbite all pose potentially lethal dangers that must be conveyed quickly [3].
Yet not all these signals feel the same or even relay the same levels of risk. Eating spicy food might hurt, but it won’t kill you. Additionally, the onset of pain doesn’t necessarily relay its danger [3]. Stepping on a tack instantly causes a shooting pain that runs from your heel to your spinal cord, while generalized lower back pain may be less acutely painful but can still cause lifelong problems.
Pain is different from other sensations and environmental signals because the way it feels is easily changed by other stimuli. One initial theory of why pain can change in intensity is called the gate control theory, discovered in 1965 by two early neuroscience pain researchers, Wall and Melzack [4]. This theory states that both painful (nociceptive) and non-painful (non-nociceptive) sensory signals are transmitted to the spinal cord from their respective nerve fibers. If the painful signals outweigh the neutral signals, the nociceptive sensation is transmitted to the brain and the person feels pain. Conversely, the gate control theory states that if the “gate” or neutral sensations outweigh the painful ones, the amount of pain a person experiences is diminished [4]. This theory aims to explain why rubbing or massaging a spot in pain can sometimes help eliminate the pain. For example, holding your side (the “gate” in this case) can eliminate a cramp (the pain), or rubbing a sore muscle can alleviate discomfort [5, 6].
Still, significant questions remain as to why people experience the same pain differently. The gate control theory doesn’t explain why two people might experience the same stimulus (for example, a broken ankle), yet have completely different perceptions of pain [5]. Additionally, the gate control tactic has limitations and doesn’t seem to ever block out pain entirely, only alleviate some of the intensity [5].
Part of the answer to why people feel pain in different ways lies in arguably one of the most discussed drug targets in the brain: opioid receptors [5]. Known primarily for their media focus in the past two decades, opioids are a class of drugs prescribed for intense pain relief. The drugs also cause feelings of relaxation and euphoria, inciting a high potential for abuse. Opioid receptors are the proteins these drugs bind to, which set off a chain of events in the spinal cord and brain to decrease pain. Though the exogenous (external) drugs attract more public attention, opioid receptors, and some forms of opioids themselves, are endogenous, meaning they are naturally produced by the body in response to stimuli like a broken arm or twisted ankle. Endogenous opioids don’t cause the same off-target effects as synthetic opioids like morphine because they are delivered directly to the targeted sites by immune cells, while synthetic opioids act anywhere opioid receptors can be found. This explains why morphine might make you feel euphoric, but a broken ankle doesn’t suddenly cause delirious relaxation [5]. There are three main kinds of endogenous opioid receptors: the mu (μ) opioid receptor, the delta (δ) opioid receptor, and the kappa (κ) opioid receptor, which all bind different types of opioid peptides in the spinal cord and cortex [7].
Though exogenous opioids have made headlines in the past two decades as drugs of potential pharmaceutical abuse, endogenous opioid receptors are involved in many different processes, such as emotional regulation, immune function, respiratory and cardiovascular systems, and even trigger hibernation in some mammals [8]. In the context of pain relief, synthetic opioids like morphine, fentanyl, and oxycodone work because they enhance the same systems that are already present in the body [8]. For example, one study showed that levels of β-endorphin, an opioid peptide that binds to the mu receptor, increased in the blood following muscle injury or dangerous infection, indicating that an injury causes these endogenous opioids to be released [9]. The study stated that binding between the naturally occurring β-endorphin and the mu receptor (which also binds fentanyl and morphine, and is blocked by naloxone) induced analgesia, or pain relief [9].
Similar studies have also indicated that endogenous opioid receptors are needed for natural pain regulation. Mice without mu opioid receptors are overly sensitive to heat, while those without delta opioid receptors experience more intense mechanical pain like pressure or pinching [7]. Additionally, mice without kappa opioid receptors exhibit stronger reactions to triggers of visceral, or internal pain [7]. In another study, dental patients who were given naloxone, which blocks endogenous mu opioid receptor binding, showed similar results [5].
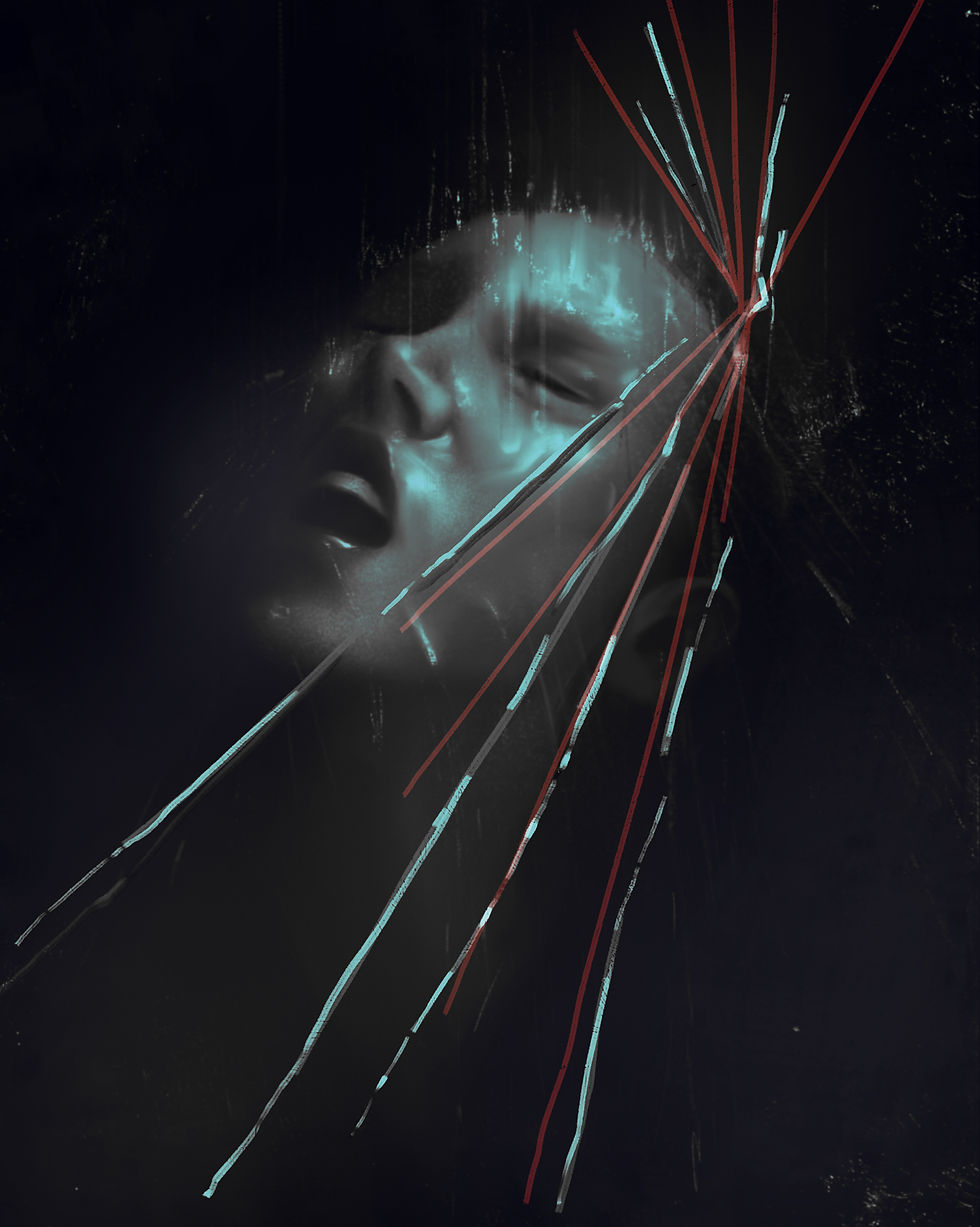
These patients, whose mu opioid receptors could not bind endorphins, experienced significantly more pain than those given a placebo. Essentially, blocking the binding of natural opioids to their receptors increased pain because it prevented the body from responding to the pain [5].
Despite this understanding that endogenous opioids do exist and block pain, the opioid system is exceptionally tricky to understand. For one, opioid application doesn’t always relieve pain [10]. One study revealed that while low levels of exogenous morphine application resulted in decreased pain responses in mice, high doses of morphine increased pain responses, indicative through behaviors such as scratching, biting, and licking. Furthermore, these symptoms were not reversed by naloxone, which blocks opioids from binding to their receptors and often used to reverse opioid overdose. In humans, high levels of exogenous opioids, such as in someone abusing drugs like oxycontin, have been linked to a heightened level of pain, sudden muscle contractions, and pain in response to non-painful stimuli like light touch. With opioids, there is such a thing as “too much of a good thing.” Opioid overuse causes far more problems than it solves [10].
Still, it is not well understood why small doses of opioids are extremely helpful in reducing pain, while high doses cause the exact opposite effect [11]. The current theory posits that morphine not only binds to mu opioid receptors that inhibit pain, but it also binds to other excitatory receptors like glutamatergic and NMDA receptors. Excitatory receptors “excite” other parts of the body and brain instead of inhibiting, leading in some cases to more pain. These receptors are partially responsible for the “off-target effects.” When our bodies naturally produce opioids, we produce precisely the right amount to bind only to opioid receptors; when we add opioids exogenously, there is less control over where they go. Thus, at higher concentrations, morphine is more likely to set off a chain of events that carry painful signals to the brain, rather than preventing pain [11].
So how does pain modulation look in practice? One of the best ways to examine how endogenous opioids can change the way we feel pain is through the lens of one of the most common health issues in America: back pain. According to one study, as many as 70-85% of people report back pain at some point in their lifetime, and a sizable percentage report chronic pain, called Chronic Non-Specific Back Pain (CNBP) [3]. A separate study found that when people who reported CNBP received a painful stimulus, their brains showed lower levels of mu opioid receptor activation in the thalamus. This means that some people with chronic pain demonstrate difficulty activating these inhibitory systems, which are supposed to prevent painful signals from reaching the brain. Instead, such individuals experience amplified pain signals, and often develop a secondary chronic condition. It is not clear if this inability to activate mu opioid receptors is a factor of chronic pain, the cause of chronic pain, or some circular combination of both. Regardless, the study provides some insight into why people with CNBP feel pain more intensely than those without it [3].
One thing, however, is clear: treating back pain with exogenous opioids, the way doctors did throughout the 2010s, tends to be more dangerous than beneficial [3]. If patients cannot bind opioids to their mu opioid receptors in the spinal cord, adding more chemicals that attempt to bind to the same receptor does not solve the problem. Rather, it creates new problems, including the potential for addiction. Therefore, one chemical theory for why people feel pain in different ways has to do with their ability to activate opioid receptors via endogenous opioids in the nervous system. If someone cannot bind endogenous opioids, which are released in response to trauma, then they are likely to experience more intense pain and decreased pain tolerance [3].
The overlap between chronic pain and overall pain tolerance also provides an interesting model to study the way chemical signals and mental signals connect. One study, also focusing on chronic pain, found that individuals with anxiety disorders, including post-traumatic stress disorder (PTSD), generalized anxiety disorder (GAD), panic disorder (PD), and/or social anxiety disorder (SAD), showed a strong co-occurrence of chronic pain [12]. One potential explanation is that rather than anxiety causing chronic pain or vice versa, both disorders help maintain each other in what is called a “mutual maintenance model.” For example, in the instance of PTSD, distress and anxiety can remind a person of physical pain, which provokes a physical response to the trauma. Another theory asserts that anxiety disorders create a “lower threshold for alarm,” meaning that someone with an anxiety disorder is more likely to experience a strong stress response with a lower-level trigger. The bodily changes caused by arousal, such as high blood pressure, fast heart rate, quick breathing, and tense muscles, can be destructive when prolonged, in the same way that chronic pain provides consistent activation of systems that are only meant for short-term activation. When in a state of stress, the body responds by decreasing the rates of digestion, immune responses, and muscle regeneration. This overlap describes one component of what we might call pain tolerance, in which the base level of how anxiety activates a stress response plays a big role in how a person might tolerate physical pain [12].
In a similar vein, pain catastrophizing, or stressing about anticipated pain, can also impact how strongly one experiences pain. One study suggested that the main predictor of reported pain was how painful the subject expected the experience to be [13]. This expectation then creates a self-fulfilling prophecy. Take, for example, getting a flu shot at the doctor. If you expect the shot to be extremely painful, your body will respond accordingly; your muscles will tense, you might wince in expectation, or you experience an increased heart rate. Since you anticipate the shot will be painful, you’re more likely to experience increased pain, which in turn will validate your expectation of pain. This feedback loop will solidify the belief that shots are very painful, and the next time you get a shot, you’ll be reminded of how painful it was the time before, and so on. A different person may have the same biological response of beta-endorphins and opioid receptors, but lower expectations of pain, which will cause them to experience less physical pain during and after the shot. Expectations exacerbate pain intensity, which in turn changes pain tolerance [13].
This theory of pain catastrophizing was replicated in another study. Here, the researchers told subjects to place their hands in cold water [14]. Before the task, half of the subjects were told that the task might be dangerous and lead to nerve damage, while the other half was informed that the task would not result in any kind of injury. The first group exhibited significantly higher anxiety before the task, and that increased anxiety caused
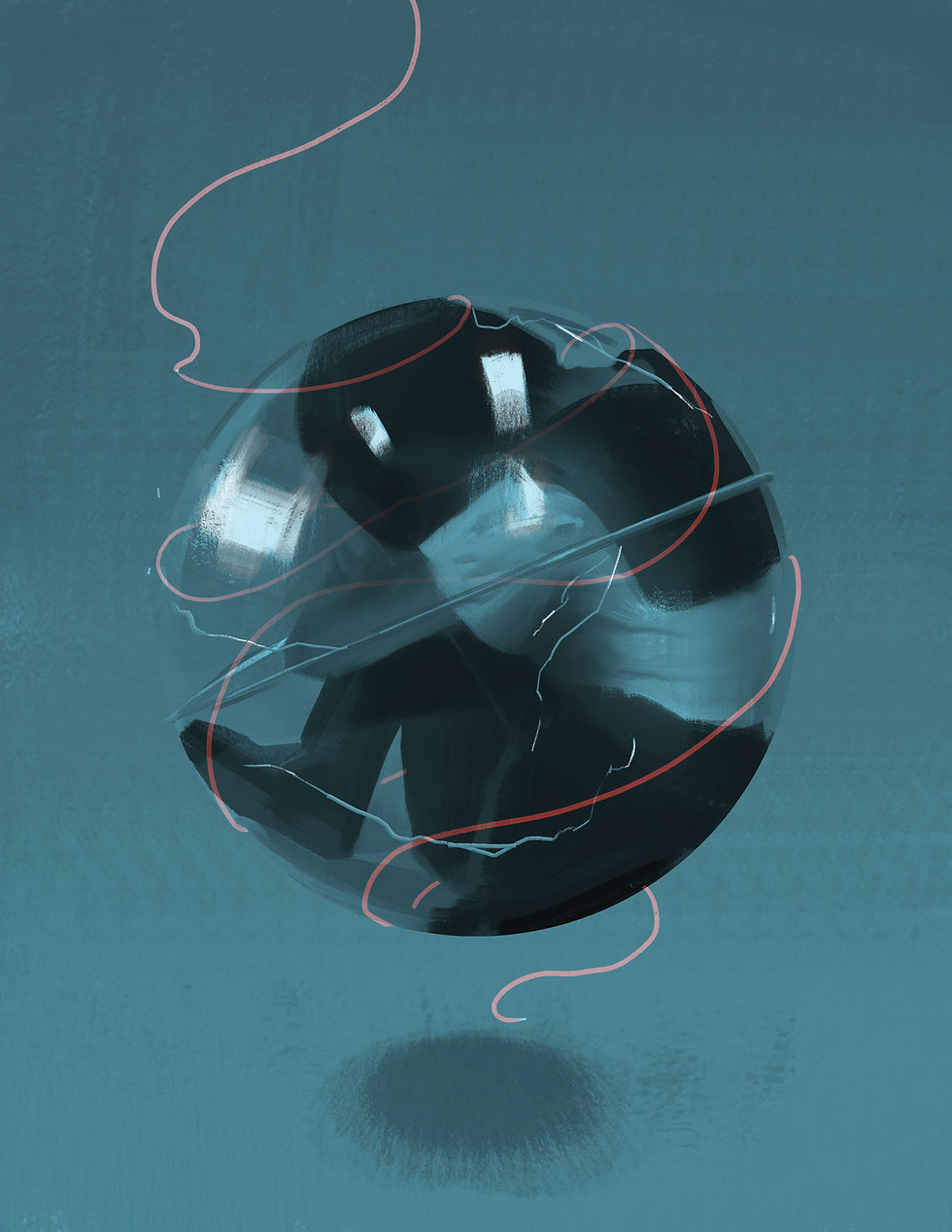
more intense feelings of pain. In contrast, the second group did not report increased anxiety and found the task to be less painful [14].
So why does your hand hurt more than your friend’s when you both trip and fall on the pavement? The answer is complicated. Your level of chronic pain and endogenous opioids play a role, and your anxiety and mental state might impact the feeling. Or, perhaps as you were falling towards the concrete, all you could think was “this is going to hurt so much.” Neuroscience research has only scratched the surface of how we feel pain and why it is so unreliable. All we know is that the systems that convey pain from our fingertips to our brains are modulated in many different places along the way, changing the overall sensation and the way we interpret it. Pain is unpleasant, and humans spend so much time, money, and effort avoiding it. Ironically, however, pain is necessary to live. It’s a warning sign, sending rapid signals to your brain that say “hey, this thing might kill you, let’s not try it again.” It’s an inconsistent signal, and an imperfect one, but it exists for a reason: pain keeps us alive.
REFERENCES:
Nagasako, E. M., Oaklander, A. L., & Dworkin, R. H. (2003). Congen- ital insensitivity to pain: an update. Pain, 101(3), 213–219. https://doi. org/10.1016/S0304-3959(02)00482-7
Institute of Medicine (US) Committee on Pain, Disability, and Chronic Illness Behavior. (1987). Pain and Disability: Clinical, Behavioral, and Public Policy Perspectives. (M. Osterweis, A. Kleinman, & D. Mechanic, Eds.). Washington (DC): National Academies Press (US). Retrieved from http://www.ncbi.nlm.nih.gov/books/NBK219254/
Martikainen, I. K., Peciña, M., Love, T. M., Nuechterlein, E. B., Cum- miford, C. M., Green, C. R., ... Zubieta, J.-K. (2013). Alterations in endogenous opioid functional measures in chronic back pain. The Journal of Neuroscience: The Official Journal of the Society for Neuroscience, 33(37), 14729–14737. https://doi.org/10.1523/JNEUROSCI.1400-13.2013
Melzack, R., & Wall, P. D. (1965). Pain mechanisms: a new theory. Science (New York, N.Y.), 150(3699), 971–979. https://doi.org/10.1126/sci- ence.150.3699.971
Kirkpatrick, D. R., McEntire, D. M., Hambsch, Z. J., Kerfeld, M. J., Smith, T. A., Reisbig, M. D., ... Agrawal, D. K. (2015). Therapeutic Basis of Clin- ical Pain Modulation. Clinical and Translational Science, 8(6), 848–856. https://doi.org/10.1111/cts.12282
Lee, H.-K., & Kirkwood, A. (2019). Mechanisms of Homeostatic Synaptic Plasticity in vivo. Frontiers in Cellular Neuroscience, 13. https://doi. org/10.3389/fncel.2019.00520
Feng, Y., He, X., Yang, Y., Chao, D., Lazarus, L. H., & Xia, Y. (2012). Current Research on Opioid Receptor Function. Current Drug Targets, 13(2), 230–246.
Corder, G., Castro, D. C., Bruchas, M. R., & Scherrer, G. (2018). Endog- enous and Exogenous Opioids in Pain. Annual review of neuroscience, 41, 453–473. https://doi.org/10.1146/annurev-neuro-080317-061522
Molina, P. E. (2002). Stress-specific opioid modulation of haemodynamic counter-regulation. Clinical and Experimental Pharmacology & Physiolo- gy, 29(3), 248–253. https://doi.org/10.1046/j.1440-1681.2002.03638.x
Watanabe, C. (2014). Mechanism of Spinal Pain Transmission and Its Regulation. Yakugaku Zasshi, 134(12), 1301–1307. https://doi. org/10.1248/yakushi.14-00203
Sakurada, T., Komatsu, T., & Sakurada, S. (2005). Mechanisms of nocicep- tion evoked by intrathecal high-dose morphine. Neurotoxicology, 26(5), 801–809. https://doi.org/10.1016/j.neuro.2004.12.011
Asmundson, G. J. G., & Katz, J. (2009). Understanding the co-occurrence of anxiety disorders and chronic pain: state-of-the-art. Depression and Anxiety, 26(10), 888–901. https://doi.org/10.1002/da.20600
Baker, S. L., & Kirsch, I. (1991). Cognitive mediators of pain percep- tion and tolerance. Journal of Personality and Social Psychology, 61(3), 504–510. https://doi.org/10.1037//0022-3514.61.3.504
Cimpean, A., & David, D. (2019). The mechanisms of pain tolerance and pain-related anxiety in acute pain. Health Psychology Open, 6(2), 2055102919865161. https://doi.org/10.1177/2055102919865161
Comments